The biology of hair color and marking in mice.
About a hundred years ago, the Mus Musculus was only known in its wild form, nowadays called the “agouti” color variety. Today, Mus Musculus can be found in up to 130 variations, from red to blue to tricolor. But how does such a thing happen? To better understand this, we dive deep into the biological matter and principles below. Most of this has become known through years of research in mice. But almost all the knowledge gained in this way can also be applied to other (small) rodents.
Melanin and the melanocyte
A single hair consists of 3 parts. In a longitudinal section (Figure 1A) it can be seen that the outer part consists of the hair scales.
Below that is the bark, mainly this part of the hair contains the pigment that plays an important role, the so-called melanin.
The medulla is located in the central part of the hair.
The formation and growth of the hair takes place in the hair follicle (Figure 1B).
At the bottom of this hair follicle are rapidly dividing cells from which the so-called keratinocytes arise, these cells form the final keratin from which the hair arises. The growth area also contains the melanocytes, these whimsically shaped cells make the melanin, which is transferred to the hair-forming keratinocytes via their offshoots.
The melanocyte is a specialized cell that determines the final hair color through its production of melanin.
This article will discuss how the melanocytes are formed, how they get to their place in the body, how the dyes are made in special organelles of the melanocyte and how these dyes are then transferred to the hair for its final use. to give coloring.
Each sub-process in this long road is determined by a group of genes designated for it (Table 1).
It is important to know that these genes not only contribute to the formation of hair color, but also have other functions in the cell.
For example, these genes mutually influence each other and this can result in the fact that certain color mutations can be associated with other, more harmful abnormalities.
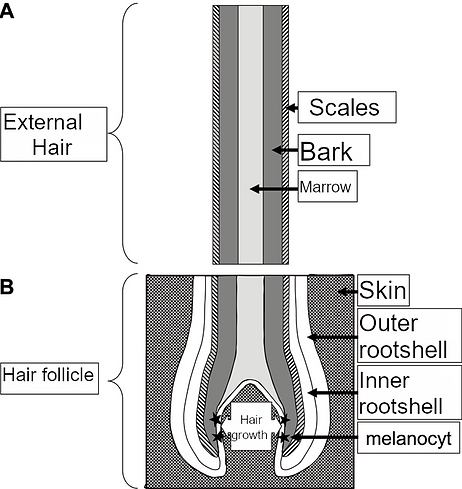
Figure 1
Schematic representation of the longitudinal section of a hair (a) and the associated hair follicle (b). Hair growth takes place at the bottom of the hair follicle and the pigment is formed in the melanocyte.
The origin of the melanocyte
During early embryonic development, an invagination occurs on the back in the outer cell layer in the developing embryo, during a process called neurulation, a tubular structure is formed (Figure 2). This process creates the neural tube, which will later develop into the central nervous system. A group of cells called the neural crest cells develop on either side of this tube, these cells do not all stay in this place but spread throughout the developing embryo. A number of very important structures later grow from these neural crest cells. For example, large parts of the skull originate from these neural crest cells, but cells of the inner ear also originate from the neural crest cells. All melanocytes that give the hair their color are also formed from neural crest cells, in this phase called melanoblasts that emigrate to the hair follicles. Mutations in a number of genes involved in neural crest formation, neural crest cell development and migration belong to the class of spotting genes. Because the melanoblasts cannot end up properly in certain places, no pigment will be formed in these places later, so that the hairs remain white. White spots will also appear if the melanoblasts die prematurely or cannot multiply. Table 1 shows some genes involved in these processes. Some of these are very notorious, such as the Kit (W/White spotting) and Mitf genes. The Kit gene encodes a protein that transmits a signal from outside the cell to the cell nucleus. In the cell nucleus, the MITF transcription factor protein will then regulate the activity of a large number of other genes. Some of these are involved in cell migration, others have a direct role in pigment production. As a result, if there is a mutation in the MITF or other genes with similar processes, hair pigment can no longer be created. But serious genetic abnormalities can also arise, such as eyes that are too small and osteoporosis, after all, these processes also partly arise under the control of the MITF protein. Some mutations in Kit can lead to abnormalities in blood and germ cells, because these tissues and organs are also partly dependent on properly functioning neural crest cells.

Image 2
Schematic representation of a cross-section of an embryo, at the level of the back, during early development. The formation of melanocytes from neural crest cells that move through the embryo during development is shown.
When the melanoblast finally ends up in the right place in the skin and develops further, the pigment-producing cell called melanocyte is created there. The melanocyte is a specialized cell that can produce melanin. This happens in special vesicles in the cell, the so-called melanosomes (image 3). The production of very many cell proteins and their transport takes place in all kinds of vesicles. Proper formation of all these vesicles is therefore very important for the proper functioning of the cell and the organs and tissues of which these cells are a part. One of the proteins that is essential for the proper formation and transport of these vesicles is the so-called Rab38, better known as chocolate among breeders. The chocolate mutation leads to a malfunction of the vesicle transport, as a result of which the final melanosome is abnormal. Rab38 is also involved in the transport of various other vesicles into the cell, and impaired lung function has been observed in mice with a Rab38 mutation (chocolate). Because a substance produced by lung cells cannot be properly excreted either.

Image 3
Schematic representation of a melanocyte. In the nucleus, the genes are read, which then leads to protein production in the ER. From this point, the proteins are further transported by the Golgi and transport vesicles. Finally, the formation of the melanosome takes place in which the melanin is produced. The mature melanasome migrates to the dendrite end of the cell and passes melanin to the hair-forming keratinocyte.
There are two types of melanosomes. One type contains the enzymes involved in the formation of the red brown/black eumelanin, while the pheomelanosomes make melanin that can vary from yellow to red. The development of the melanosome is an essential and complex mechanism involving an estimated 20 different genes. When these genes do not work properly as a result of mutations, there is often too little pigment. But many of these genes are also involved in the formation of other cell vesicles, such as the vesicles responsible for the production and transport of clotting proteins. Mutations in this class of color mutations are therefore often found to cause problems with blood clotting. If the melanosome is eventually formed, disturbances in normal functions can occur. For example, the Silver protein forms structures in the early melanosome that are important for the capture of the melanin to be formed later. Other proteins that have a function in the melanosome are a series of enzymes that can convert the precursor substances into the final melanin Due to the many variations in the action of the genes involved in the formation of the melanosomes and their production of the pigments eumelanin and pheomelanosomes, many different color pigments can develop that later give the color to the coat.
Transport of the melanosome
The pigment, of whatever composition, is now made. But it’s not in the hair cell yet. The filled melanosome has to be moved from its place in the center of the cell to the edge of the cell, the so-called dendrite tip. This is done via so-called conveyor belts that are part of the cell skeleton. At the edge of the cell, the melanosome is transferred to the keratinocyte, which will form the final hair from keratin. How exactly this transfer works is largely unknown, but again a number of genes are involved that can determine the coat color. More is known about the transport of the melanosome and a number of the genes involved have now been identified. One of these genes is dilute. If this does not work properly, too many melanosomes will remain in the center of the melanocyte, instead of being transported to the dendrite tip. The result is that the melanocyte becomes darker and the hair lighter. When the dilute gene does not work properly in humans, the so-called Griscelli syndrome type 1 arises. This is characterized by gray hair and neurological abnormalities, which shows that the dilute gene not only has a function in melanocytes, but also in many other cells.
Eumelanin-Pheomelanin Exchanges
When we look at the guard hair of a wild mouse, we see a kind of band pattern. The end is black (eumelanin), then a part that is red/yellow (pheomelanin) and then the base which is black again (eumelanin). This agouti pattern arises because during the growth of the hair more eumelanin, then pheomelanin and then eumelanin is made successively in the melanocyte. The switch in the function of the two different types of melanosomes is controlled from an antenna on the cell boundary. This melanocortin receptor (e, recessive yellow) binds, among other things, the cell hormone agouti. When this happens, less eumelanin and more pheomelanin is produced, which gives a yellow color. During hair growth, first little, then more for a short time, and finally little agouti is formed in the hair follicle, causing the light band in the hair. Of course, there are also a number of genes involved in transmitting the signal from the melanocortin receptor to the relevant melanosomes. The Mahogany gene is one of them and therefore has its influence on melanin exchange.
General genes
Finally, there are genes that regulate general processes in the body, and these are also important in the production of coat color. Two of them are mottled and toxic milk. The proteins encoded by these genes are involved in the metabolism of copper in the body. Copper is part of the enzyme tyrosinase (albino), and without copper this important protein responsible for melanin production cannot function. It is therefore true that when the copper metabolism is disturbed (mottled) this also has an effect on the coat color.
Finally
From the above it becomes clear that the formation of coat color is a very complex mechanism. First, the neural crest cells are formed, which then move to the right place in the skin. The pigment-producing melanocytes then arise from these cells. The melanosomes are formed in these cells, which produce the final eumelanin and pheomelanin. These pigments are then transported to the tip of the melanocyte where they are transferred to the hair-forming keratinocyte. During all these steps, different genes are active, all of which have their own influence during the different phases of hair color formation. We have also seen that many of these genes are needed during all kinds of other processes in different organs and tissues. As a result, certain coat colors are often accompanied by other, sometimes serious and sometimes more subtle, deviations. As a breeder it is good to always keep this in mind.

Table 1: Overview of a number of characterized genes involved in coat color formation.
Sources:
D.C. Bennett, M.L. Lamoreux. The color loci of mice – a genetic century. Pigment Cell Research 2003;16:333-344.
E.N. Marieb, J. Mallatt. Human anatomy. Benjamin/Cummings Publishing Company, Redwood City, CA, USA, 1992
K. Osanai, R Oikawa, J Higuchi, M Kobayashi, K Tsuchihara, M Iguchi, H Jongsu, H Toga, DR Voelker. A mutation in Rab38 small GTPase causes abnormal lung surfactant homeostasis and aberrant alveolar structure in mice. Amercan Journal of Pathology 2008;173:1265-1274.
M.V. Schiaffino. Signaling pathways in melanosome biogenesis and pathology. The International Journal of Biochemistry & Cell Biology 2010;42:1094-1104.
Y. Shimomura, A.M. Christiano. Biology and genetics of hair. The Annual Reviews of Genomics and Human Genetics. 2010;11:109-132.
W.K. Silvers. The coat colors of mice. Springer Verlag, New York, 1979.
E. Steingrimsson, N.G. Copeland, N.A. Jenkins. Mouse coat color mutations: from fancy mice to functional genomics. Developmental Dynamics 2006;235:2401-2411.